HTML Full Text
INTRODUCTION
The World Health Organization (WHO) is an international organization responsible for maintaining the highest level of health and well-being. As per the WHO, microorganisms are one of the challenging threats that are affecting global health, exponentially. Antimicrobial resistance of modern medicine is a continuously growing crisis accredited because of their overuse or misapplication of these medications. In addition, economic burden and challenging regulatory requirements are the biggest lacking reasons that are obstacles to the development of new drugs in the pharmaceutical industry. Over the years, bacterial resistance to the existing pharmaceuticals has continuously increased, thus to minimize resistance and maximize the biological effectiveness of antimicrobial agents, it is necessary to explore the mechanism of antibacterial resistance through another biomolecular approach and negate the effectiveness of the therapeutic drug (Alshibl et al., 2020; Breijyeh et al., 2020).
Staphylococcus aureus (S. aureus), Bacillus subtilis (B. subtilis), Escherichia coli (E coli), and Pseudomonas aeureginosa (P. aeruginosa) are the most frequent etiological agents of bacteremia, the bacterial pathogens which are responsible for several ailments and even shut down the immune system of the body system. However, some of the common risk factors from such pathogens are neutropenia, cystic fibrosis, pneumonia, septicemia, and severe burns (Yayan et al., 2015).
To evade such pathogenic microorganisms, it has become a challenging endeavor to the emerging healthcare system worldwide. These strains resist a wide class of existing antibacterial drugs such as fluoroquinolones, β-lactam antibiotics, glycopeptides, macrolides, and oxazolidinones (Fair and Tor, 2014).
However, the risk factors for an individual’s morbidity and mortality are continuously increasing due to such pathogenic microbes. Pneumonia is one of the severe diseases caused by the transmission of P. aeruginosa in healthcare centres or even through medical sinks, disinfectants, equipment, and food. It has become a serious problem due to the difficult elimination of P. aeruginosa as it is resistant to many antibiotics such as penicillin G, aminopenicillin, β-lactamase inhibitors, cephalosporins (first generation), piperacillin, cefepime, aminoglycosides, carbapenems, colistin, etc. (Bush and Bradford, 2016). Therefore, it is important for choosing the right antibiotic to alleviate the trends in resistance of P. aeruginosa (Akter et al., 2014). In addition, E. coli is a Gram-negative bacteria which is well recognized as a part of the normal intestinal flora; in case the strength becomes disbalance, it causes severe life-threatening sequelae (Bobak and Guerrant, 2014).
Coumarins are naturally and synthetically processed organic compounds and their derivatives have been poeticised for several past years for the treatment of microbial infection. Coumarins are acknowledged with excellent therapeutic action as antioxidant, anti-inflammatory, anticoagulant, antifungal, anticancer, and antibacterial activity (Pereira et al., 2018). Because of their noteworthy biological importance, the synthesis of new coumarins derivatives is being assessed continuously as antibacterial and anti-inflammatory and are applied as modern therapy against the drugs which show antibacterial resistance (Lin et al., 2012). Several synthesized coumarin derivatives have been explored to their pharmacological potential, and many of them have exhibited potential biological activity such as antibacterial and antifungal. The attention to such important compounds has been increased significantly because it is suggested that these compounds work against several viral infections such as HIV activity, etc. Furthermore, potential cytostatic activity is being exhibited by coumarin derivatives and therefore can be deliberated for anticancer therapy as potential candidates. Recently, coumarin derivatives of dimer and tetramer type were reported to possess HIV-1 integrase inhibitory activity. These facts urged the researchers to commence the synthesis of coumarin derivatives and explore their biological potential (López-Rojas et al., 2018).
The fascinating properties of different coumarins can be attributed to their chemical reactivity as 2H-chromen-2-one core; its aromatic ring comprised a series of hydrophobics, π–π, CH–π, and cation–π interactions and the two oxygen atoms in the lactone ring can hydrogen-bond to a sequence of amino acid remains in different classes of enzymes and receptors. Furthermore, the double bond in the lactone provides assistance to create the planar system and consents for charge delocalization between the aromatic ring and the carbonyl group of the lactone which confers the characteristic fluorescence of this class of compounds (Stefanachi et al., 2018). Considering the facts, the present aim of the study is associated with synthesis and exploration of 4-anilinocoumarin derivatives as a potential antimicrobial agent against the drugs-causing antibacterial resistance, even the development of a new antibacterial agent would facilitate an effective and economic antibacterial drug.
MATERIALS AND METHODS
Chemicals and microbial strains
Thin-layer chromatography (TLC) silica gel 60 F254 (Merck KGaA, 64271 Darmstadt, Germany), CDCl3 as the solvent, TMS as an internal standard, S. aureus (MTCC No. 3161), B. subtillis (MTCC No. 441), E. coli (MTCC NO. 1687), and P. aeruginosa (MTCC NO. 424) and the reagents, solvents, and catalysts were of analytical grade and used directly for the analysis.
Chemistry
The synthesis process was accomplished in four steps: in the first step, compound 1a was prepared via reaction of aniline with 4-hydroxycoumarin. Furthermore, this compound was treated with ethylchloroacetate in the presence of KOH/K2CO3, yielding an N-alkylated product (2a). The reaction of this product with hydrazine hydrate yielded desired hydrazide compound (3a). Schiff base derivatives (4a–4j) were obtained by reaction of 3a with different aromatic aldehydes in the presence of glacial acetic acid in alcohol. The percentage purity of the compounds was checked by TLC using appropriate solvent systems where a single spot was considered the indication of a completed reaction. The structures of all the synthesized derivatives were elucidated by mass, IR, and 1HNMR spectroscopical analysis. The steps included in the synthesis are described below.
Synthesis of 4-anilino-2H-1-benzopyran-2-one(1a’)
A mixture of 4-hydroxycoumarin (0.01 mol) and aniline (0.01 mol) was stirred at 160°C for 20 minutes. The resulting mixture was dissolved in methanol (30 ml) and then 0.1 M aq. NaOH was added dropwise with stirring. After 5–10 minutes, the precipitate formed was washed, dried, and recrystallized. The completion of the reaction was confirmed by TLC using ethyl acetate and benzene as a mobile system.
Synthesis of ethyl [(2-oxo-2H-1-benzopyran-4-yl)(phenyl)amino]acetate (2a’)
4-anilino-2H-1-benzopyran-2-one (0.01 mol) was treated with ethylchloroacetate (0.01 mol) in the presence of KOH/K2CO3 and dry DMF (70 ml) was heated at 92°C–94°C with stirring for 4 hours. After that, the solution was poured into ice water; the precipitate was filtered, washed with water, and dried to yield the N-substituted compound. The completion of the reaction was confirmed by TLC using acetone and n-hexane as a mobile system.
Synthesis of 2-[(2-oxo-2H-1-benzopyran-4-yl)(phenyl)amino] acetohydrazide (3a’)
Compound 3’ was reacted with hydrazine (0.01 mol) in presence of ethanol and refluxed for 2 hours. The completion of the reaction was confirmed by TLC and the yield of the compound.
Synthesis of Schiff bases (4a’–4o’)
Compound 4’ (0.01 mol) was reacted with a different aromatic aldehyde (0.01 M) in the presence of glacial acetic acid in alcohol and refluxed for 2–4 hours to yield corresponding Schiff bases. The steps involved in chemistry for the synthesis of Schiff base coumarin derivatives are shown in Figure 1.
Spectroscopical analysis of synthesized compounds
Mass spectrometric (MS) analysis
The MS analysis was carried out to identify the synthesis compounds based on their molecular mass obtained from the MS spectral data. The MS system is attached with Water’s ACQUITY UPLC(TM) system (Waters Corp., MA) and adjoined with an auto-sampler, binary solvent delivery system, column manager, and MS detector. In brief, 1 mg/ml concentration were prepared in LCMS grade solvent for each sample; acetonitrile (A: 85%) and water (B: 15%) were used as chromatographic solvent run throughout a monolithic capillary silica-based C18 column [ACQUITY UPLC(R) BEH C18 1.7 µm, 2.1 × 100 mm]; the injection volume for the sample was set at 2 µl. The flow rate of the nebulizer gas and cone gas was set at 500 l/hours and 50 l/hours, respectively. In mass analysis, electrospray ionization (ESI) was used as the ion source and the temperature for mass source was fixed at 120°C, while capillary and cone voltage was set at 3.0 and 40 KV, respectively. For collision, argon was active at a pressure of 5.5 × 10−5 torr. The obtained spectral data were interpreted and tentatively identified (Gaurav et al., 2020).
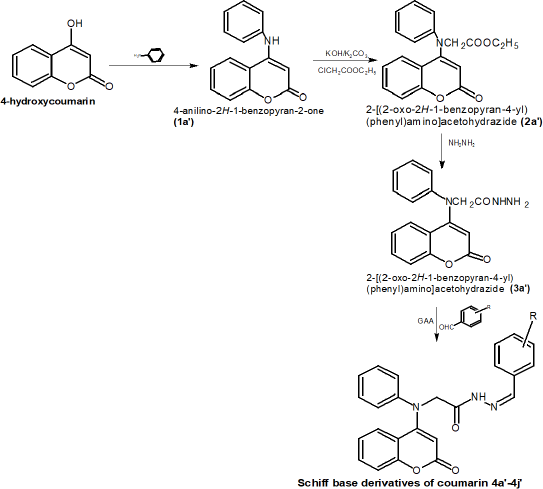 | Figure 1. Schematic representation of the reaction process for the synthesis of Schiff base coumarin derivatives.
[Click here to view] |
FT-IR spectroscopy analysis of isolated compounds
The spectral analysis for synthesized compounds was carried out by using Win-IR, Bio-Rad FTS spectrophotometer. In brief, each sample was mixed with potassium bromide individually and proceeded ahead for spectroscopical analysis in the range of 4,000–400 cm−1 (Hosseini et al., 2019).
1H-NMR spectroscopic analysis of isolated compounds
NMR spectroscopical analysis of synthesized compounds was carrid out as per the described method using Bruker Avance 500 MHz NMR spectrometer. Each sample (5 mg) was dissolved in deuterated methanol (CD3OD) as an NMR solvent, while tetramethylsilane was used as an internal standard. The samples proceeded for spectroscopic analysis by NMR technique (Prasad and Sati, 2011).
Antibacterial activity
In vitro antibacterial activity of the synthesized coumarin derivatives compounds was conducted through zone inhibition assay/well-diffusion assay against S. aureus, B. subtilis, E. coli, and P. aeureginosa bacterial strains using the standard protocol with some modification (Kumar et al., 2020). In brief, the bacterial inoculum from each secondary strain culture (prepared from 100 μl of primary suspension containing approx.1 × 108 CFU/ml pathological tested bacteria) was evenly spread over the surface of the agar petri dish plates using a sterile cotton swab. Thereafter, four holes of 5 mm diameter were made using a sterile tip. 10 µl of drug solution (1 mg/ml) was added into the wells and the controls well were treated with 10 µl of autoclaved double-distilled water which was considered the control treatment. Plates were incubated for 72 hours under aerobic conditions and at 37°C temperature. The antimicrobial effect was determined by the clear zone in the agar, which was measured after the completion of treatment.
RESULTS AND DISCUSSION
Chemistry
During the synthesis process, 10 coumarin derivatives were synthesized through Schiff base reaction and characteristically monitored by TLC to evaluate that there was no by-products formed. The completion of the reaction was checked by the TLC method. The percentage yield and melting points of each synthesized compound were determined. The resulted outcomes of the study are summarized in Table 1.
Spectroscopic analysis of synthesized compounds
In spectroscopic analysis, MS, FT-IR, and 1HNMR analyses were conducted for the characterization of synthesized coumarin derivatives. The predicted common coumarin derivative structure with atom numbering is shown in Figure 2.
2-[(2-oxo-2H-1-benzopyran-4-yl)(phenyl)amino]-N’-[(E)-phenylmethylidene] acetohydrazide (4a’)
MS (ESI) m/z: 397.46 (M+1); (FTIR, υmax, cm−1 ); 3,378.59, 3,315.11 (N/NH), 3,112.07 (CH2), 1,735.99 (C=C/C=N), 1,650.43 (C=O), 1,583.01 (diketones), 1,492.48 (C-O-C), 1,339.97 (CH), 1,145.42, 1,087.35, 989.88, and 723.39 (aromatic, aliphatic amines, and aromatic C-H vibrations). 1H-NMR (CD3OD, 500MHz): δ 10.473, 8.421 (2H, 2s, NH, CH-16), 8.348 (1H, m, Ar, CH-5), 7.952 (2H, m, Ar, CH-2’, 6’), 7.762, and 7.612, (4H, 2m, Ar, CH-7, Ar, CH-3’, 4’, 5’), 7.372 (2H, m, Ar, CH-6, 8), 7.037, 6.692, and 6.502 (5H, 3m, Ar, Aniline), 5.112 and 3.942 (3H, 2s, Ar, CH-3, CH2).
2-[(2-oxo-2H-1-benzopyran-4-yl)(phenyl)amino]-N’-[(E)-(4-chlorophenyl)methylidene] acetohydrazide (4b’)
MS (ESI) m/z: 432.07 (M+1); (FTIR, υmax, cm−1); 3,455.70, 3,347.62 (=N/-NH), 3,042.91(CH2), 1,722.95 (C=C/C=N), 1,638.17 (C=O), 1,473.29, and 1,357.36 cm−1 (C-O-C and monosubstituted alkynes), 1,135.01, 1,023.55, 1,087.35, 989.88, and 725.83 (aromatic, aliphatic amines, and aromatic C-H and C-Cl). 1H-NMR (CD3OD, 500MHz): δ 10.473 and 8.421 (2H, 2s, NH, CH-16), 8.346 (1H, m, Ar, CH-5), 7.952 (2H, d, J=6.74 Hz, Ar, CH-2’, 6’), 7.752 (1H, m, Ar, CH-7), 7.332 (4H, m, Ar, CH-6, 7, CH-3’, 5’), 7.021, 6.695, and 6.537 (5H, 3m, Ar, Aniline), 5.664 and 3.925 (3H, 2s, Ar, CH-3, CH2).
2-[(2-oxo-2H-1-benzopyran-4-yl)(phenyl)amino]-N’-[(E)-(3-chlorophenyl)methylidene] acetohydrazide (4c’)
MS (ESI) m/z: 431.78 (M+1); (FTIR, υmax, cm−1); 3,421.85, 3,318.74 (=N/-NH), 3,107.21 (CH2), 1,635.32 (C=O), 1,553.77, 1,419.99, 1,335.48 (diketones, C-O-C, aromatic C-H, and mono-substituted alkynes), 1,093.48, 1,001.37, 830.95, and 759.32 (aromatic, aliphatic amines and aromatic C-H, C-Cl vibrations). 1H-NMR (CD3OD, 500MHz): δ 10.473 and 8.421 (2H, 2s, NH, CH-16), 8.346 (1H, m, Ar, CH-5), 7.632 and 7.385 (7H, m, Ar, CH-6, 7, 8; CH-2’, 4’, 5’, 6’), 7.023, 6.68, and 6.515 (5H, 3m, Ar, Aniline), 5.676 and 3.937 (3H, 2s, Ar, CH-3, CH2).
(E)-N’-(3-aminobenzylidene)-2-((2-oxo-2H-chromen-4-yl)(phenyl)amino)acetohydrazide (4d’)
MS (ESI) m/z: 412.58 (M+1); (FTIR, υmax, cm−1); 3,421.29 (=N/-NH), 3,053.01 (CH2), 1,647.13 (C=O), 1,543.55, 1,413.93, and 1,329.17 (C-O-C, aromatic C-H, and monosubstituted alkynes), 1,125.36, 1,007.22, and 847.28 cm−1 (aromatic, aliphatic amines, and aromatic C-H vibrations). 1H-NMR (CD3OD, 500MHz): δ 10.473 and 8.421 (2H, 2s, NH, CH-16), 8.346 (1H, m, Ar, CH-5), 7.751 (1H, m, Ar, CH-7), 7.275 (3H, m, Ar, CH-6, 8; CH-5’), 7.275, 7.025, 6.901, 6.794, 6.695, and 6.539 (8H, 6m, Ar, aniline and aminobenzylidene), 5.672, 5.377, and 3.926 (5H, 3s, Ar, CH-3, NH2, CH2).
2-[(2-oxo-2H-1-benzopyran-4-yl)(phenyl)amino]-N’-[(E)-(4-hydroxyphenyl)methylidene ]acetohydrazide (4e’)
MS (ESI) m/z: 413.64 (M+1); (FTIR, υmax, cm−1); 3,397.07 (=N/-NH), 3,355.58 (OH), 2,981.23 (CH2), 1,648.13 (C=O), 1,523.55, and 1,405.68 cm−1 (C-O-C, aromatic C-H / monosubstituted alkynes), 1,183.95, 1,063.79, 1,007.02, and 739.74 (aromatic, aliphatic amines, and aromatic C-H vibrations). 1H-NMR (CD3OD, 500MHz): δ 10.473, 9.521, and 8.421 (3H, 3s, NH, Ar-OH, CH-16), 8.346 (1H, m, Ar, CH-5), 7.752 (3H, m, Ar, CH-7, CH-2’, 6’), 7.385 (2H, m, Ar, CH-6, 8), 7.155, 6.901, 6.804, and 6.521 (7H, 4m, Ar, aniline, and phenol), 5.672 and 3.926 (3H, 2s, Ar, CH-3, CH2).
2-[(2-oxo-2H-1-benzopyran-4-yl)(phenyl)amino]-N’-[(E)-(3,4-dihydroxyphenyl) methylidene]acetohydrazide (4f’)
MS (ESI) m/z: 430.26 (M+1); (FTIR, υmax, cm−1); 3,463.05 (=N/-NH), 3,365.28 (OH), 3,082.75 (CH2), and 1,879.36 (C=N) 1,673.42 (C=O), 1,588.07, 1,507.19, and 1,395.53 cm−1 (C-O-C, aromatic C-H / monosubstituted alkynes), 1,068.27, 1,007.25, 827.53, and 791.39 cm−1 (aromatic, aliphatic amines, and aromatic C-H vibrations). 1H-NMR (CD3OD, 500MHz): δ 10.473, 9.521, and 8.421 (4H, 3s, NH, OH, CH-16), 8.346 (1H, m, Ar, CH-5), 7.752 (1H, m, Ar, CH-7), 7.395 (2H, m, Ar, CH-6, 8), 7.175, 7.051, 6.752, and 6.536 (8H, 4m, Ar, aniline, and phenol), 5.672 and 3.926 (3H, 2s, Ar, CH-3, CH2).
2-[(2-oxo-2H-1-benzopyran-4-yl)(phenyl)amino]-N’-[(E)-(4-dimethylaminophenyl) methylidene]acetohydrazide (4g’)
MS (ESI) m/z: 440.82 (M+1); (FTIR, υmax, cm−1); 3,341.16 (=N/-NH), 3,006.72 and 2,968.83 (CH2/ CH3), 1,639.49 (C=O). 1,507.33, 1,439.05, and 1,396.47 (C-O-C, aromatic C-H / monosubstituted alkynes), 1,078.11, 1,006.25, 783.91, and 654.32 (aromatic, aliphatic amines, and aromatic compounds cis- and trans-substitution stretching vibrations). 1H-NMR (CD3OD, 500MHz): δ 10.473 and 8.421 (2H, 2s, NH, CH), 8.346 (1H, m, Ar, CH-5), 7.752 (1H, m, Ar, CH-7), 7.395 (4H, m, Ar, CH-6, 8; CH-2’, 6’), 7.155, 6.891, 6.702, and 6.526 (7H, 3m, Ar, aniline, and dimethylamino-benzylidene), 5.772, 3.926, and 2.893 represent nine protons (9H, 3s, Ar, CH-3, CH2, 2CH3).
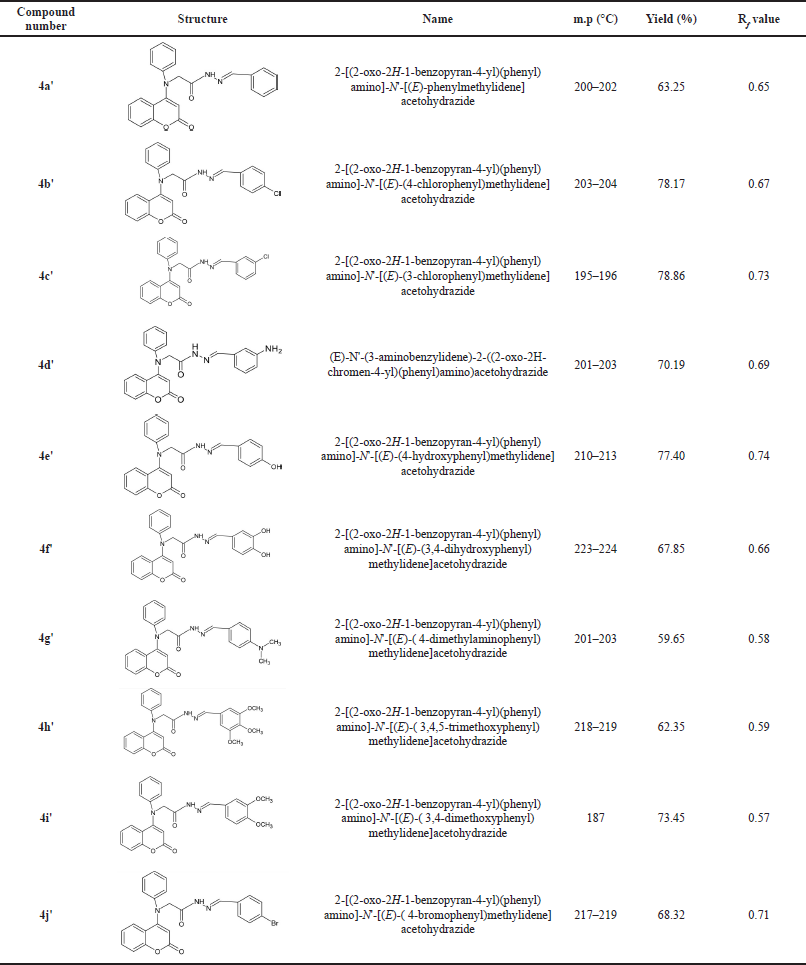 | Table 1. Chemical structures/IUPAC name and physicochemical characters of synthesized compounds.
[Click here to view] |
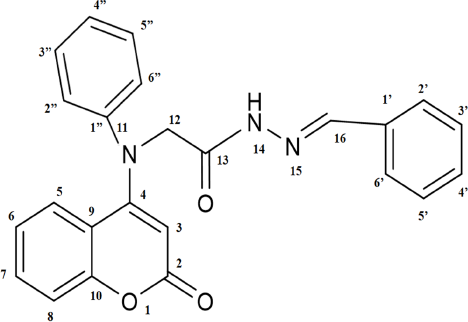 | Figure 2. Predicted common structure of coumarin derivative with atomic numbering.
[Click here to view] |
2-[(2-oxo-2H-1-benzopyran-4-yl)(phenyl)amino]-N’-[(E)-(3,4,5-trimethoxyphenyl) methylidene]acetohydrazide (4h’)
MS (ESI) m/z: 487.34 (M+1); (FTIR, υmax, cm−1); 3,427.62 (=N/-NH), 3,001.02, 2,965.22 and 1,681.05 (CH2/CH3 and C=O), 1,560.77, 1,501.08, 1,424.89, and 13,47.26 (diketones, C-O-C, aromatic C-H / monosubstituted alkynes), 1,058, 979.43, and 777.35 (aromatic, aliphatic amines, and aromatic compounds cis- and trans-substitution stretching vibrations). 1H-NMR (CD3OD, 500MHz): δ 10.473 and 8.421 (2H, 2s, NH, CH), 8.346 (1H, m, Ar, CH-5), 7.752 (1H, m, Ar, CH-7), 7.395 (2H, m, Ar, CH-6, 8), 7.211 (2H, s, Ar, CH-2’, 6’), 7.114, 6.813, and 6.532 (5H, 2m, Ar-aniline), 5.672, 3.936, 3.846, and 3.873 (12H, 4s, Ar, CH-3, CH2, 3CH3).
2-[(2-oxo-2H-1-benzopyran-4-yl)(phenyl)amino]-N’-[(E)-(3,4-dimethoxyphenyl) methylidene]acetohydrazide (4i’)
MS (ESI) m/z: 458.06 (M+1); (FTIR, υmax, cm−1); 3,345.11 (=N/-NH), 3,019.45, 2,929.05 (-CH2/CH3), 1,663 (C=O), 1,632.14, 1,527.33, 1,472.28, and 1,376.77 (diketones, C-O-C, aromatic C-H / monosubstituted alkynes), 1,198.46, 1,117.25, and 864 (aromatic, aliphatic amines, and C-H stretching vibrations). 1H-NMR (CD3OD, 500MHz): δ 10.473 and 8.387 (2H, 2s, NH, CH), 8.335 (1H, m, Ar, CH-5), 7.762 (1H, m, Ar, CH-7), 7.413 (3H, m, Ar, CH-2; CH-6, 8), 7.191 and 6.873 (2H, 2m, Ar, CH-5’, 6’), 7.014, 6.703, and 6.532 (5H, 3m, Ar-aniline), 5.672, 3.925 and 3.716 (9H, 3s, Ar, CH-3, CH2, CH3).
2-[(2-oxo-2H-1-benzopyran-4-yl)(phenyl)amino]-N’-[(E)-( 4-bromophenyl)methylidene] acetohydrazide (4j’)
MS (ESI) m/z: 476.01 (M+1); (FTIR, υmax, cm−1); 3,391.15 (=N/-NH), 3,089.47 (CH2), 1,721.35 (C=O), 1,539.92, 1,412.73 and 1,358.06 (diketones, C-O-C, aromatic C-H / monosubstituted alkynes) 1,099.63, 900.37, and 702.93 (aromatic, aliphatic amines, C-H and C-Br stretching vibrations). 1H-NMR (CD3OD, 500MHz): δ 10.473 and 8.387 (2H, 2s, NH, CH-16), 8.335 (1H, m, Ar, CH-5), 7.755 represents five proton (5H, m, Ar, CH-7, bromobenzylidene), 7.392 (2H, m, Ar, CH-6, 8), 7.163, 6.801, and 6.532 (5H, 2m, Ar-aniline), 5.672 and 3.925 (3H, 2s, Ar, CH-3, CH2).
The structures of synthesized compounds with the melting point, percentage yield, and TLC retention factors (Rf) are summarized in Table 1.
Antibacterial activity of compounds
In vitro antibacterial activity of the synthesized Schiff base coumarin derivatives was carried out through zone inhibition assay/well-diffusion assay against S. aureus, B. subtilis, E. coli, and P. aeureginosa bacterial strain. The inhibitory action to bacterial strain was considered with the direct proportion of obtained zone observed in the agar plate. The resulted data revealed that compounds 4a’, 4c’, 4d’, 4g’, and 4h’ were showing good activity, while other compounds exhibited lower activity against the targeted strains of microorganism. The zones of inhibition for the potentially active compounds were found as follows: 5.905 ± 1.011, 5.595 ± 0.728, 6.145 ± 1.138, 5.285 ± 0.176, and 6.595 ± 0.021 mm against S. aureus; 4.82 ± 0.042, 4.6 ± 0.367, 3.97 ± 0.014, 5.25 ± 0.028, and 5.335 ± 0.021 mm against B. subtilis; 3.8 ± 0.056, 5.295 ± 0.063, 5.805 ± 0.728, 3.255 ± 0.021, and 3.755 ± 0.091mm against E. coli; and 5.51 ± 0.381, 5.63 ± 0.226, 5.61 ± 0.001, 5.435 ± 0.121, and 5.66 ± 0.014 mm inhibition, respectively. The resulted outcomes of the selected compounds may be the potential agents for antimicrobial activity. The outcomes of the antimicrobial study are shown in Figures 3 and 4.
DISCUSSION
Schiff bases are the ketone or aldehyde organic compounds that are abundantly used in the synthesis of several organic compounds together with coumarins. Although the classical synthesis mainly involves condensation of carbonyl compounds and is used for highly electrophilic carbonyl and nucleophilic amine compounds (Dixit et al., 2010; Ronad et al., 2008), in our study several coumarin derivatives were synthesized through a Schiff base reaction. During the synthesis process, the reaction was characteristically monitored by TLC to determine the purity of compounds. The synthesized coumarin derivatives were screened as a potential antimicrobial agent against the survivability of several B. subtilis, S. aureus, E. coli, and P. aeruginosa are considered as the major factor of several severe deleterious onsets inside the body (Ramachandran, 2014). Among the tested 10 coumarin derivatives, 3 compounds were found to exhibit significant (p < 0.05) inhibitory activity against each bacterial strain. The outcomes revealed that compounds 4a’, 4d’, and 4h’ are the potential antibacterial agents against the survivability of Gram-positive and negative bacterial strains.
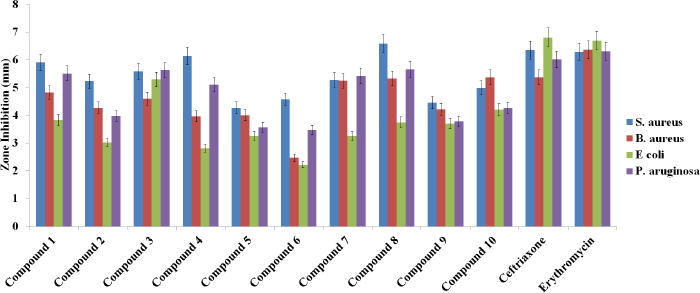 | Figure 3. A bar graph representation of the antibacterial activity of synthesized compounds evaluated through zone inhibition assay against different strains of microorganisms.
[Click here to view] |
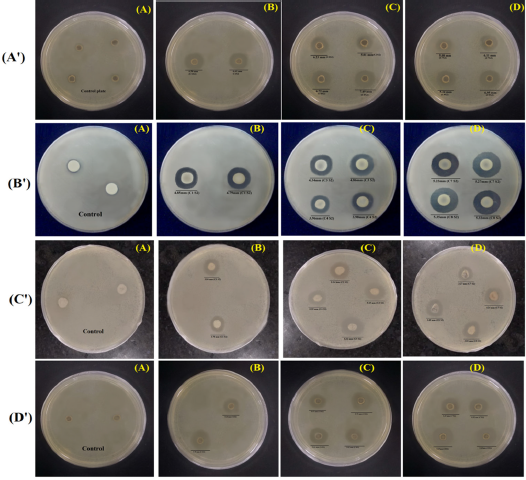 | Figure 4. Antibacterial activity of coumarins derivatives (4a’, 4c’, 4d’, 4g’, and 4h’). (A’) the control plate and drug-treated plates against S. aureus, whereas (A) shows the control plate; (B) shows the test plate of compound 4a’; and (C and D) show the test plate of compounds 4c’, 4d’, 4g’, and 4h’. (B’) shows the control plate and treated plates against B. subtilis. (A) shows the control plate; (B) shows the test plate of compound 4a’; and (C and D) show the test plate of compounds 4c’, 4d’, 4g’, and 4h’. (C’) shows the control plate and treated plates against E. coli. (A) shows the control plate; (B) shows the test plate of compound 4a’; and (C and D) show the test plate of compounds 4c’, 4d’, 4g’, and 4h’. (D’) shows the control plate and treated plates against P. aruginosa. (A) shows the control plate; (B) shows the test plate of compound 4a’; (C and D) show the test plate of compounds 4c’, 4d’, 4g’, and 4h’.
[Click here to view] |
Furthermore, the outcomes were matched with another reported study which conferred that the antimicrobial activity of coumarins is symmetrized in a diversified array in the field of pharmaceutical sciences. In a study cited by Završnik et al. (2008), it is reported that tetramer 3,3,’3’,’3’’’-(1,4-dim- ethylenphenyl)tetra (4-hydroxycoumarin) derivatives showed potential antimicrobial activity against several Gram-positive and Gram-negative bacteria such as B. subtilis, S. aureus, E. coli, and P. aeruginosa (Završnik et al., 2008). In a reported study, 45 coumarin derivatives were tested against the same bacterial strains and reported the inhibitory properties of coumarins. So far, the most effective antibacterial coumarin derivative was represented as osthenol with MIC values ranging between 125 and 62.5 µg/ml. Furthermore, the authors claimed that the synthesized coumarins are the potential candidate for antibacterial against these strains (De Souza et al., 2005). A study conducted by Kawase et al. (2001) evaluated that the substituents ester or carboxylic acids on the coumarin ring exhibits potent inhibitory activity against both several Gram-positive and negative microbial strains. In addition, the presence of phenolic hydroxyl or carboxylic acid functional group of coumarins are necessary to possess higher activity against Helicobacter pylori (Kawase et al., 2001).
A study conducted by Alshibl et al. (2020) evaluated the antioxidant, antibacterial, and anti-inflammatory activity of the several synthesized compounds. The outcomes of the study revealed that among a total series of the coumarin derivatives, Coumarin-sulfonamide compounds 8a–d confirmed with the significant antioxidant activity against DPPH free radicals, while 7c,d, 8c,d, and 9c,d unveiled antimicrobial activity almost equal or greater than the antimicrobials against used as standards for tested microorganism. Because of the anti-inflammatory evaluation, pyranocoumarins and coumarin-sulfonamide compound 9a exhibited more significant antiproteinase activity than aspirin. Furthermore, the authors claimed that five compounds exhibited the potent activity than aspirin. In vivo outcomes for evaluation of anti-inflammatory activity assessed pharmacologically on formaldehyde-induced rat paw edema and revealed potent inhibitory activity against the induced edema. In addition, pyranocoumarin derivative 5a and coumarin-sulfonamide derivative 8d were found more active toward COX-2 isozyme (Alshibl et al., 2020).
Therefore, it can be demonstrated that coumarins derivative is the most potential candidate as an antioxidant, anti-inflammatory, and antibacterial agent. In addition, the outcome from the present study revealed coumarin derivatives as another alternative for antimicrobial activity. The developed coumarins derivatives can be assisted as auspicious candidates for more potent and highly effective antimicrobial agents.
CONCLUSION
The present study enlightens that among the series of different synthesized 4-anilinocoumarin derivatives, compounds 4a’, 4d’, and 4h’ are the most potent antimicrobial agents against Gram-positive and negative bacterial strains which exhibit significant even higher antibacterial effect than the standard drugs. In addition, it can be demonstrated that the synthesized coumarin derivatives cover those antimicrobial agents that shows resistance even restricted to provide significant therapeutic effect.
ACKNOWLEDGMENT
The authors thank Professor Vijay Bhalla, Principal, SGT College of Pharmacy, SGT University, Gurugram-122505, India, for providing necessary facilities.
AUTHOR CONTRIBUTIONS
All authors made substantial contributions to conception and design, acquisition of data, or analysis and interpretation of data; took part in drafting the article or revising it critically for important intellectual content; agreed to submit to the current journal; gave final approval of the version to be published; and agree to be accountable for all aspects of the work. All the authors are eligible to be an author as per the international committee of medical journal editors (ICMJE) requirements/guidelines.
FUNDING
There is no funding to report.
CONFLICTS OF INTEREST
The authors report no financial or any other conflicts of interest in this work.
ETHICAL APPROVALS
This study does not involve experiments on animals or human subjects.
DATA AVAILABILITY
All data generated and analyzed are included within this research article.
REFERENCES
Akter S, Shamsuzzaman SM, Jahan F. Community acquired bacterial pneumonia: aetiology, laboratory detection and antibiotic susceptibility pattern. Malays J Pathol, 2014; 36(2):97-103.
Alshibl HM, Al-Abdullah ES, Haiba ME, Alkahtani HM, Awad GE, Mahmoud AH, Ibrahim BM, Bari A, Villinger A. Synthesis and evaluation of new coumarin derivatives as antioxidant, antimicrobial, and anti-inflammatory agents. Molecules, 2020; 25(14):3251. CrossRef
Bobak DA, Guerrant RL. Nausea, vomiting, and noninflammatory diarrhea. Mandell, Douglas, and Bennett’s principles and practice of infectious diseases, 2015:1253. doi: 10.1016/B978-1-4557-4801-3.00100-4 CrossRef
Breijyeh Z, Jubeh B, Karaman R. Resistance of Gram-negative bacteria to current antibacterial agents and approaches to resolve it. Molecules, 2020; 25(6):1340. CrossRef
Bush K, Bradford PA. β-Lactams and β-lactamase inhibitors: an overview. Cold Spring Harb Perspect Med, 2016; 6(8):a025247. CrossRef
De Souza SM, Delle Monache F, Smânia A. Antibacterial activity of coumarins. Zeitschrift fuer Naturforschung C, 2005; 60(9-10):693–700. CrossRef
Dixit RB, Uplana RA, Patel VA, Dixit BC, Patel TS. Development and evaluation of cefadroxil drug loaded biopolymeric films based on chitosan-furfural schiff base. Sci Pharma, 2010; 78(4):909–26. CrossRef
Fair RJ, Tor Y. Antibiotics and bacterial resistance in the 21st century. Perspect Med Chem, 2014; 6:25–64. CrossRef
Gaurav, Zahiruddin S, Parveen B, Ibrahim M, Sharma I, Sharma S, Sharma AK, Parveen R, Ahmad S. TLC-MS bioautography-based identification of free-radical scavenging, α-Amylase, and α-Glucosidase inhibitor compounds of antidiabetic tablet BGR-34. ACS Omega, 2020; 5(46):29688–97. CrossRef
Hosseini SM, Abbasalipourkabir R, Jalilian FA, Asl SS, Farmany A, Roshanaei G, Arabestani MR. Doxycycline-encapsulated solid lipid nanoparticles as promising tool against Brucella melitensis enclosed in macrophage: a pharmacodynamics study on J774A. 1 cell line. Antimicrob Resist Infect Control, 2019; 8(1):1–2. CrossRef
Kawase M, Varu B, Shah A, Motohashi N, Tani S, Saito S, Debnath S, Mahapatra S, Dastidar SG, Chakrabarty AN. Antimicrobial activity of new coumarin derivatives. Arzneimittelforschung, 2001; 51(01):67–71. CrossRef
Kumar V, Ain S, Kumar B, Ain Q, Gaurav. Optimization and evaluation of topical gel containing solid lipid nanoparticles loaded with luliconazole and its anti-fungal activity. Int J Pharm Res, 2020; doi:10.31838/ijpr/2020.SP2.169
Lin PY, Yeh KS, Su CL, Sheu SY, Chen T, Ou KL, Lin MH, Lee LW. Synthesis and antibacterial activities of novel 4-hydroxy-7-hydroxy-and 3-carboxycoumarin derivatives. Molecules, 2012; 17(9):10846–63. CrossRef
López-Rojas P, Janeczko M, Kubi?ski K, Amesty Á, Mas?yk M, Estévez-Braun A. Synthesis and antimicrobial activity of 4-substituted 1, 2, 3-triazole-coumarin derivatives. Molecules, 2018; 23(1):199. CrossRef
Pereira TM, Franco DP, Vitorio F, Kummerle AE. Coumarin compounds in medicinal chemistry: some important examples from the last years. Curr Topics Med Chem, 2018; 18(2):124–48. CrossRef
Prasad DW, Sati SP. A new 2, 8-dihydroxy-1, 6-dimethoxy xenthones from Cythula tomesntosa. Oriental J Chem, 2011; 27(2):765–7.
Ramachandran G. Gram-positive and gram-negative bacterial toxins in sepsis: a brief review. Virulence, 2014; 5(1):213–8. CrossRef
Ronad P, Dharbamalla S, Hunshal R, Maddi V. Synthesis of novel substituted 7-(Benzylideneamino)-4-Methyl-2H-Chromen-2-one derivatives as anti-inflammatory and analgesic agents. Arch Pharm, 2008; 341(11):696–700. CrossRef
Stefanachi A, Leonetti F, Pisani L, Catto M, Carotti A. Coumarin: a natural, privileged and versatile scaffold for bioactive compounds. Molecules, 2018; 23(2):250. CrossRef
Yayan J, Ghebremedhin B, Rasche K. Antibiotic resistance of Pseudomonas aeruginosa in pneumonia at a single university hospital center in Germany over a 10-year period. PLoS One, 2015; 10(10):e0139836. CrossRef
Završnik D, Muratovi? S, Špirtovi? S, Softi? D, Medi?-Šari? M. The synthesis and antimicrobial activity of some 4-hydroxycoumarin derivatives. Bosn J Basic Med Sci, 2008; 8(3):277. CrossRef